E. Dillon & Company
- Swords Creek
- 800-234-8970
To log into the website, simply start Minecraft with the latest version of LabyMod and click on your head in the Minecraft main menu or in the LabyMod settings. If you have issues starting Minecraft with LabyMod or you cannot find the head in your main menu, make sure that you have the latest version of LabyMod or use our alternative way and join the Minecraft server auth.labymod.net. Default Keybinds (15) Note: Keybinds are slightly different depending on your Minecraft version. Check the Wurst Wiki for details.
If you have questions about specific products or services we provide, please don't hesitate to contact us.
INTRODUCTION
Cracks in buildings and building materials normally result from restrained movement. This movement may originate within the material, as with volume changes due to moisture loss or acquisition, temperature expansion or contraction, or may result from movements of adjacent or supporting materials, such as deflection of beams or slabs. In many cases, movement is inevitable and must be accommodated or controlled.
Designing for effective crack control requires an understanding of the sources of stress which may cause cracking. It would be a simple matter to prevent cracking if there were only one variable. However, prevention is made more difficult by the fact that cracking often results from a combination of sources.
CAUSES OF CRACKING
There are a variety of potential causes of cracking. Understanding the cause of potential cracking allows the designer to incorporate appropriate design procedures to control it. The most common causes of cracking in concrete masonry are shown in Figure 1 and are discussed below.
Figure 1 – Proper Design Can Avert Cracking of These Types
Shrinkage/Restraint
Cracking resulting from shrinkage can occur in concrete masonry walls because of drying shrinkage, temperature fluctuations, and carbonation. These cracks occur when masonry panels are restrained from moving.
Drying Shrinkage
Concrete products are composed of a matrix of aggregate particles coated by cement which bonds them together. Once the concrete sets, this cementitious-coated aggregate matrix expands with increasing moisture content and contracts (shrinks) with decreasing moisture content. Drying shrinkage is therefore a function of change in moisture content.

Although mortar, grout, and concrete masonry units are all concrete products, unit shrinkage has been shown to be the predominate indicator of the overall wall shrinkage principally due to the fact that it represents the largest portion of the wall. Therefore, the shrinkage properties of the unit alone are typically used to establish design criteria for crack control.
For an individual unit, the amount of drying shrinkage is influenced by the wetness of the unit at the time of placement as well as the characteristics and amount of cementitious materials, the type of aggregate, consolidation, and curing. Specifically, drying shrinkage is influenced in the following ways:
- walls constructed with 'wet' units will experience more drying shrinkage than drier units ;
- increases in cement content increase drying shrinkage;
- aggregates that are susceptible to volume change due to moisture content will result in increased shrinkage; and
- units that have undergone at least one drying cycle will not undergo as much shrinkage in subsequent drying cycles (ref. 7).
Typical drying shrinkage coefficients range from 0.0002 to 0.00045 in./in. (mm/mm) or 0.24 to 0.54 in. (6.1 to 13.7 mm) in 100 ft (30.48 m).
Temperature Changes
Concrete masonry movement has been shown to be linearly proportional to temperature change. The coefficient of thermal movement normally used in design is 0.0000045 in./in./°F (0.0000081 mm/mm/°C) (ref. 2). Actual values may range from 0.0000025 to 0.0000055 in./in./°F (0.0000045 to 0.0000099 mm/ mm/°C) depending mainly on the type of aggregate used in the unit. The actual change in temperature is, of course, determined by geographical location, wall exposure, and color.
Music - hearts of iron iv: sabaton soundtrack vol. 2 download for mac. As an example, a wall constructed during 70°F (21°C) weather and subjected to a minimum temperature of 0°F (-18°C) results in a shortening of about 0.38 in. (9.7 mm) in a 100 foot (30.48 m) long wall using the 0.0000045 in./in./°F (0.0000081 mm/mm/°C) coefficient.
Carbonation
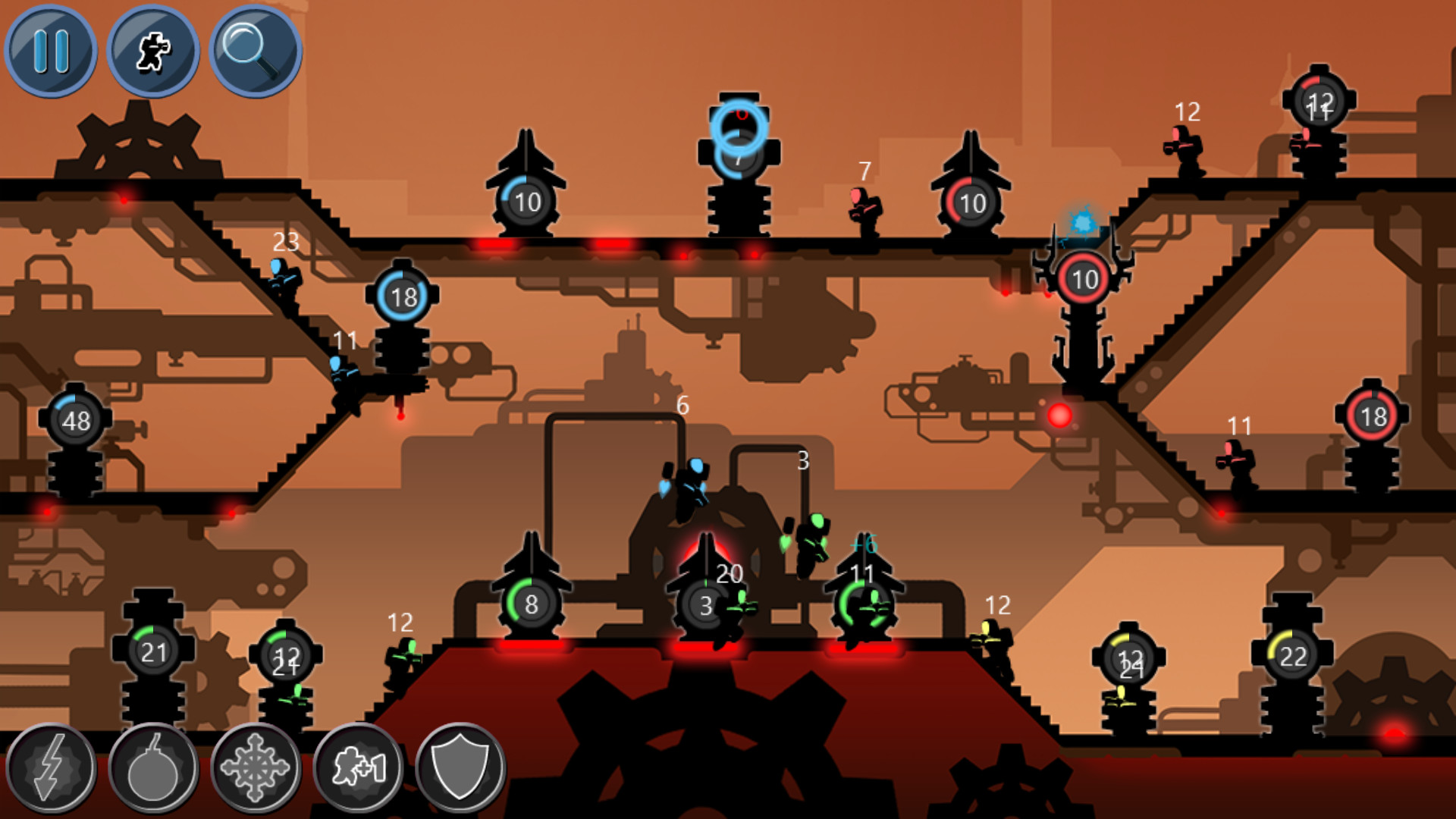
Carbonation is an irreversible reaction between cementitious materials and carbon dioxide in the atmosphere that occurs slowly over a period of several years. Since there currently is no standard test method for carbonation shrinkage, it is suggested that a value of 0.00025 in./in. (mm/mm) be used. This results in a shortening of 0.3 in. (7.6 mm) in a 100 foot (30.48 m) long wall.
Restraint
As previously mentioned, the above phenomenon produce movement in the wall. When external restraint is provided that resists this movement, the result is tension within the wall and a corresponding potential for cracking. Typically, concrete masonry walls are restrained along the bottom of the wall (mainly by the foundation) with partial restraint along the top of the wall. The ends of the typical concrete masonry wall panel may be partially restrained by pilasters or wall intersections, but this partial restraint usually does not significantly alter the wall's cracking potential. Exceptions to the typical restraint condition include cantilevered walls which are restrained along their base, but free (unrestrained) at the top. It is conservative to base general crack control design criteria on a condition of restraint along the top and bottom of the wall.
Differential Movement
Various building materials may react differently to changes in temperature, moisture, or structural loading. Any time materials with different properties are combined in a wall system, a potential exists for cracking due to differential movement. With concrete masonry construction, two materials in particular should be considered: clay brick and structural steel.
Differential movement between clay brick and concrete masonry must be considered when the two are attached since concrete masonry has an overall tendency to shrink while clay brick masonry tends to expand. These differential movements may cause cracking, especially in composite construction and in walls that incorporate brick and block in the same wythe.
Composite walls are multi-wythe walls designed to act structurally, as a single unit in resisting applied loads. The wythes are typically bonded together using wall ties at prescribed intervals to assure adequate load transfer. When the composite wall includes a clay brick wythe bonded to a concrete masonry wythe, ladder-type joint reinforcement, or box ties are used to provide some degree of lateral movement between wythes. In addition, expansion joints are installed in the clay brick wythe to coincide with control joints in the concrete masonry wythe.
When clay brick is used as an accent band in a concrete masonry wall, or vice-versa, the differential movement of the two materials may result in cracking unless provisions are made to accommodate the movement. To reduce cracking, slip planes between the band and the surrounding wall, horizontal reinforcement or more frequent control joints or a combination thereof can be used to control cracking. See Crack Control for Concrete Brick and Other Concrete Masonry Veneers (ref. 6) for more information on these approaches.
Thermal movement differences also need to be taken into consideration when using masonry in conjunction with structural steel. In addition to differences in thermal coefficients, steel shapes typically have a much higher surface area to volume ratio and tend to react to changes in temperature more quickly. This is normally accommodated with slotted and flexible connections. Concrete Masonry Walls for Metal Buildings (ref. 5) provides more detailed information on this subject.
Excessive Deflection
As walls and beams deflect under structural loads, cracking may occur. Additionally, deflection of supporting members can induce cracks in masonry elements. To reduce the potential for cracking, the following alternatives are available:
- adding reinforcing steel into the masonry to cross the expected cracks and to limit the width of the cracks,
- limiting the deflection of members providing vertical support of unreinforced masonry to acceptable levels (less than or equal to l/600 nor more than 0.3 in. (7.6 mm) due to dead load and live load when supporting unreinforced masonry) (ref. 2), and;
- utilizing movement joints to effectively panelize the masonry so that it can articulate with the deflected shape of the supporting member.
Structural Overload
All wall systems are subject to potential cracking from externally applied design loads due to wind, soil pressure or seismic forces. Cracking due to these sources is controlled by applying appropriate structural design criteria such as allowable stress design or strength design. These criteria are discussed in detail in Allowable Stress Design of Concrete Masonry and Strength Design Provisions for Concrete Masonry (refs. 1 and 9).
Settlement
Differential settlement occurs when portions of the supporting foundation subside due to weak or improperly compacted foundation soils. Foundation settlement typically causes a stair-step crack along the mortar joints in the settled area as shown in Figure 1. Preventing settlement cracking depends on a realistic evaluation of soil bearing capacity, and on proper footing design and construction.
Dragon age origins korcari wilds map. Footings should be placed on undisturbed native soil, unless this soil is unsuitable, weak, or soft. Unsuitable soil should be removed and replaced with compacted soil, gravel, or concrete. Similarly, tree roots, construction debris, and ice should be removed prior to placing footings. Adding reinforcement in foundations can also lessen the effects of differential settlement.
CRACK CONTROL STRATEGIES
In addition to the proper design strategies discussed above for structural capacity and differential movement, the following recommendations can be applied to limit cracking in concrete masonry walls.
Material Properties
Traditionally, crack control in concrete masonry has relied on specifying concrete masonry units with a low moisture content, using horizontal reinforcement, and using control joints to accommodate movement. Prior to the 2000 edition of ASTM C90 (ref. 8), low moisture content was specified by requiring a Type I moisture controlled unit. The intent was to provide designers an assurance of units with lower moisture content to minimize potential shrinkage cracking. However, there are several limitations to relying on moisture content alone since there are other factors that influence shrinkage which are not accounted for by specifying a Type I unit. Additionally, Type I units were not always inventoried by concrete masonry manufacturers. Most importantly, Type I units needed to be kept protected until placed in the wall, which was proven to be difficult on some projects. Because of the above problems associated with the Type I specification, ASTM removed the designations of Type I, Moisture-Controlled Units and Type II, Nonmoisture Controlled Units from the standard.
Due to removal of the unit type designations from ASTM C90, two methods of determining control joint spacings have been devised irrespective of unit type: 1). Empirical crack control criteria which is based on successful, historical performance over many years in various geographic conditions and 2). Engineered crack control criteria based on a Crack Control Coefficient (CCC) that includes the combined effects of movement due to drying shrinkage, carbonation shrinkage, and contraction due to temperature change. The first is presented in NCMA TEK 10-2C, Control Joints for Concrete Masonry Walls – Empirical Method (ref. 4) and the second in TEK 10-3 Control Joints for Concrete Masonry Walls – Alternative Engineered Method (ref. 3). The empirical method is the most commonly used method and is applicable to most conventional building types. The engineered method is generally used only when unusual conditions are encountered such as dark colored units in climates with large temperature swings.
Control Joints
Control joints are essentially vertical separations built into the wall to reduce restraint and permit longitudinal movement. Because shrinkage cracks in concrete masonry are an aesthetic rather than a structural concern, control joints are typically only required in walls where shrinkage cracking may detract from the appearance or where water penetration may occur. TEK 10-2C (ref. 4) provides much more detailed information on control joint details, types and locations.
Reinforcement to Limit Crack Width
In addition to external restraint, reinforcement causes some internal restraint within the wall. Reinforcement responds to temperature changes with corresponding changes in length; however, reinforcement does not undergo volumetric changes due to moisture changes or carbonation. Consequently, as the wall shrinks, the reinforcement undergoes elastic shortening (strain) which results in compressive stress in the steel. Correspondingly, the surrounding masonry offsets this compression by tension. At the point when the masonry cracks and tries to open, the stress in the reinforcement turns to tension and acts to limit the width of the crack by holding it closed.
The net effect is that reinforcement controls crack width by causing a greater number (frequency) of cracks to occur. As the horizontal reinforcement ratio (cross-sectional area of horizontal steel vs. vertical cross-sectional area of masonry) increases, crack width decreases. Smaller sized reinforcement at closer spacings is more effective than larger reinforcement at wider spacings, although horizontal reinforcement at spacings up to 144 in. (3658 mm) is considered effective in controlling crack widths in some areas.
Studies have shown that reinforcement, either in the form of joint reinforcement or reinforced bond beams, effectively limits crack width in concrete masonry walls. As indicated previously, as the level of reinforcement increases and as the spacing of the reinforcement decreases, cracking becomes more uniformly distributed and crack width decreases. For this reason, a minimal amount of horizontal reinforcement is needed when utilizing the NCMA recommended maximum control joint spacings (refs. 3 & 4).
Walls in high seismic areas with a relatively large amount of horizontal reinforcement may not require control joints, as the reinforcement alone reduces the width of shrinkage cracks to a size that can be treated effectively with water repellent coatings. Experience has shown that this can be accomplished in walls with at least 0.2% of horizontal reinforcement (ref. 3). See Table 1 for the size and spacing of reinforcement to meet this criteria.
Table 1—Maximum Spacing of Horizontal Reinforcement to Meet the Criteria As > 0.002An
References
- Allowable Stress Design of Concrete Masonry Based on the 2012 IBC & 2011 MSJC, TEK 14-7C. National Concrete Masonry Association, 2013.
- Building Code Requirements for Masonry Structures, ACI 530-05/ASCE 6-05/TMS 402-05. Reported by the Masonry Standards Joint Committee, 2005.
- Control Joints for Concrete Masonry Walls – Alternative Engineered Method, TEK 10-3. National Concrete Masonry Association, 2003.
- Control Joints for Concrete Masonry Walls – Empirical Method, TEK 10-2C. National Concrete Masonry Association, 2010.
- Concrete Masonry Walls for Metal Buildings, TR-149. National Concrete Masonry Association, 1996.
- Crack Control for Concrete Brick and Other Concrete Masonry Veneers, TEK 10-4. National Concrete Masonry Association, 2001.
- Measuring Shrinkage of Concrete Block – A Comparison of Test Methods, E.L. Saxer and H.T. Toennies, Pages 988-1004, 1957.
- Standard Specification for Loadbearing Concrete Masonry Units, ASTM C90-03. ASTM International, 2003.
- Strength Design Provisions for Concrete Masonry, TEK 14-4B. National Concrete Masonry Association, 2008.
NCMA TEK 10-01A, Revised 2005.
NCMA and the companies disseminating this technical information disclaim any and all responsibility and liability for the accuracy and the application of the information contained in this publication.
E. Dillon & Company
- Swords Creek
- 800-234-8970
If you have questions about specific products or services we provide, please don't hesitate to contact us.
INTRODUCTION
Cracks in buildings and building materials normally result from restrained movement. This movement may originate within the material, as with volume changes due to moisture loss or acquisition, temperature expansion or contraction, or may result from movements of adjacent or supporting materials, such as deflection of beams or slabs. In many cases, movement is inevitable and must be accommodated or controlled.
Designing for effective crack control requires an understanding of the sources of stress which may cause cracking. It would be a simple matter to prevent cracking if there were only one variable. However, prevention is made more difficult by the fact that cracking often results from a combination of sources.
CAUSES OF CRACKING
There are a variety of potential causes of cracking. Understanding the cause of potential cracking allows the designer to incorporate appropriate design procedures to control it. The most common causes of cracking in concrete masonry are shown in Figure 1 and are discussed below.
Figure 1 – Proper Design Can Avert Cracking of These Types
Shrinkage/Restraint
Cracking resulting from shrinkage can occur in concrete masonry walls because of drying shrinkage, temperature fluctuations, and carbonation. These cracks occur when masonry panels are restrained from moving.
Drying Shrinkage
Concrete products are composed of a matrix of aggregate particles coated by cement which bonds them together. Once the concrete sets, this cementitious-coated aggregate matrix expands with increasing moisture content and contracts (shrinks) with decreasing moisture content. Drying shrinkage is therefore a function of change in moisture content.
Although mortar, grout, and concrete masonry units are all concrete products, unit shrinkage has been shown to be the predominate indicator of the overall wall shrinkage principally due to the fact that it represents the largest portion of the wall. Therefore, the shrinkage properties of the unit alone are typically used to establish design criteria for crack control.
For an individual unit, the amount of drying shrinkage is influenced by the wetness of the unit at the time of placement as well as the characteristics and amount of cementitious materials, the type of aggregate, consolidation, and curing. Specifically, drying shrinkage is influenced in the following ways:
- walls constructed with 'wet' units will experience more drying shrinkage than drier units ;
- increases in cement content increase drying shrinkage;
- aggregates that are susceptible to volume change due to moisture content will result in increased shrinkage; and
- units that have undergone at least one drying cycle will not undergo as much shrinkage in subsequent drying cycles (ref. 7).
Typical drying shrinkage coefficients range from 0.0002 to 0.00045 in./in. (mm/mm) or 0.24 to 0.54 in. (6.1 to 13.7 mm) in 100 ft (30.48 m).
Temperature Changes
Concrete masonry movement has been shown to be linearly proportional to temperature change. The coefficient of thermal movement normally used in design is 0.0000045 in./in./°F (0.0000081 mm/mm/°C) (ref. 2). Actual values may range from 0.0000025 to 0.0000055 in./in./°F (0.0000045 to 0.0000099 mm/ mm/°C) depending mainly on the type of aggregate used in the unit. The actual change in temperature is, of course, determined by geographical location, wall exposure, and color.
As an example, a wall constructed during 70°F (21°C) weather and subjected to a minimum temperature of 0°F (-18°C) results in a shortening of about 0.38 in. (9.7 mm) in a 100 foot (30.48 m) long wall using the 0.0000045 in./in./°F (0.0000081 mm/mm/°C) coefficient.
Carbonation
Carbonation is an irreversible reaction between cementitious materials and carbon dioxide in the atmosphere that occurs slowly over a period of several years. Since there currently is no standard test method for carbonation shrinkage, it is suggested that a value of 0.00025 in./in. (mm/mm) be used. This results in a shortening of 0.3 in. (7.6 mm) in a 100 foot (30.48 m) long wall.
Restraint
As previously mentioned, the above phenomenon produce movement in the wall. When external restraint is provided that resists this movement, the result is tension within the wall and a corresponding potential for cracking. Typically, concrete masonry walls are restrained along the bottom of the wall (mainly by the foundation) with partial restraint along the top of the wall. The ends of the typical concrete masonry wall panel may be partially restrained by pilasters or wall intersections, but this partial restraint usually does not significantly alter the wall's cracking potential. Exceptions to the typical restraint condition include cantilevered walls which are restrained along their base, but free (unrestrained) at the top. It is conservative to base general crack control design criteria on a condition of restraint along the top and bottom of the wall.
Differential Movement
Various building materials may react differently to changes in temperature, moisture, or structural loading. Any time materials with different properties are combined in a wall system, a potential exists for cracking due to differential movement. With concrete masonry construction, two materials in particular should be considered: clay brick and structural steel.
Differential movement between clay brick and concrete masonry must be considered when the two are attached since concrete masonry has an overall tendency to shrink while clay brick masonry tends to expand. These differential movements may cause cracking, especially in composite construction and in walls that incorporate brick and block in the same wythe.
Composite walls are multi-wythe walls designed to act structurally, as a single unit in resisting applied loads. The wythes are typically bonded together using wall ties at prescribed intervals to assure adequate load transfer. When the composite wall includes a clay brick wythe bonded to a concrete masonry wythe, ladder-type joint reinforcement, or box ties are used to provide some degree of lateral movement between wythes. In addition, expansion joints are installed in the clay brick wythe to coincide with control joints in the concrete masonry wythe.
When clay brick is used as an accent band in a concrete masonry wall, or vice-versa, the differential movement of the two materials may result in cracking unless provisions are made to accommodate the movement. To reduce cracking, slip planes between the band and the surrounding wall, horizontal reinforcement or more frequent control joints or a combination thereof can be used to control cracking. See Crack Control for Concrete Brick and Other Concrete Masonry Veneers (ref. 6) for more information on these approaches.
Thermal movement differences also need to be taken into consideration when using masonry in conjunction with structural steel. In addition to differences in thermal coefficients, steel shapes typically have a much higher surface area to volume ratio and tend to react to changes in temperature more quickly. This is normally accommodated with slotted and flexible connections. Concrete Masonry Walls for Metal Buildings (ref. 5) provides more detailed information on this subject.
Excessive Deflection
As walls and beams deflect under structural loads, cracking may occur. Additionally, deflection of supporting members can induce cracks in masonry elements. To reduce the potential for cracking, the following alternatives are available:
- adding reinforcing steel into the masonry to cross the expected cracks and to limit the width of the cracks,
- limiting the deflection of members providing vertical support of unreinforced masonry to acceptable levels (less than or equal to l/600 nor more than 0.3 in. (7.6 mm) due to dead load and live load when supporting unreinforced masonry) (ref. 2), and;
- utilizing movement joints to effectively panelize the masonry so that it can articulate with the deflected shape of the supporting member.
Structural Overload
All wall systems are subject to potential cracking from externally applied design loads due to wind, soil pressure or seismic forces. Cracking due to these sources is controlled by applying appropriate structural design criteria such as allowable stress design or strength design. These criteria are discussed in detail in Allowable Stress Design of Concrete Masonry and Strength Design Provisions for Concrete Masonry (refs. 1 and 9).
Settlement
Differential settlement occurs when portions of the supporting foundation subside due to weak or improperly compacted foundation soils. Foundation settlement typically causes a stair-step crack along the mortar joints in the settled area as shown in Figure 1. Preventing settlement cracking depends on a realistic evaluation of soil bearing capacity, and on proper footing design and construction.
Footings should be placed on undisturbed native soil, unless this soil is unsuitable, weak, or soft. Unsuitable soil should be removed and replaced with compacted soil, gravel, or concrete. Similarly, tree roots, construction debris, and ice should be removed prior to placing footings. Adding reinforcement in foundations can also lessen the effects of differential settlement.
CRACK CONTROL STRATEGIES
In addition to the proper design strategies discussed above for structural capacity and differential movement, the following recommendations can be applied to limit cracking in concrete masonry walls.
Material Properties
Traditionally, crack control in concrete masonry has relied on specifying concrete masonry units with a low moisture content, using horizontal reinforcement, and using control joints to accommodate movement. Prior to the 2000 edition of ASTM C90 (ref. 8), low moisture content was specified by requiring a Type I moisture controlled unit. The intent was to provide designers an assurance of units with lower moisture content to minimize potential shrinkage cracking. However, there are several limitations to relying on moisture content alone since there are other factors that influence shrinkage which are not accounted for by specifying a Type I unit. Additionally, Type I units were not always inventoried by concrete masonry manufacturers. Most importantly, Type I units needed to be kept protected until placed in the wall, which was proven to be difficult on some projects. Because of the above problems associated with the Type I specification, ASTM removed the designations of Type I, Moisture-Controlled Units and Type II, Nonmoisture Controlled Units from the standard.
Control Craft 3
Due to removal of the unit type designations from ASTM C90, two methods of determining control joint spacings have been devised irrespective of unit type: 1). Empirical crack control criteria which is based on successful, historical performance over many years in various geographic conditions and 2). Engineered crack control criteria based on a Crack Control Coefficient (CCC) that includes the combined effects of movement due to drying shrinkage, carbonation shrinkage, and contraction due to temperature change. The first is presented in NCMA TEK 10-2C, Control Joints for Concrete Masonry Walls – Empirical Method (ref. 4) and the second in TEK 10-3 Control Joints for Concrete Masonry Walls – Alternative Engineered Method (ref. 3). The empirical method is the most commonly used method and is applicable to most conventional building types. The engineered method is generally used only when unusual conditions are encountered such as dark colored units in climates with large temperature swings.
Control Joints
Control joints are essentially vertical separations built into the wall to reduce restraint and permit longitudinal movement. Because shrinkage cracks in concrete masonry are an aesthetic rather than a structural concern, control joints are typically only required in walls where shrinkage cracking may detract from the appearance or where water penetration may occur. TEK 10-2C (ref. 4) provides much more detailed information on control joint details, types and locations.
Control Craft 3 Hacked
Reinforcement to Limit Crack Width
In addition to external restraint, reinforcement causes some internal restraint within the wall. Reinforcement responds to temperature changes with corresponding changes in length; however, reinforcement does not undergo volumetric changes due to moisture changes or carbonation. Consequently, as the wall shrinks, the reinforcement undergoes elastic shortening (strain) which results in compressive stress in the steel. Correspondingly, the surrounding masonry offsets this compression by tension. At the point when the masonry cracks and tries to open, the stress in the reinforcement turns to tension and acts to limit the width of the crack by holding it closed.
The net effect is that reinforcement controls crack width by causing a greater number (frequency) of cracks to occur. As the horizontal reinforcement ratio (cross-sectional area of horizontal steel vs. vertical cross-sectional area of masonry) increases, crack width decreases. Smaller sized reinforcement at closer spacings is more effective than larger reinforcement at wider spacings, although horizontal reinforcement at spacings up to 144 in. (3658 mm) is considered effective in controlling crack widths in some areas.
Studies have shown that reinforcement, either in the form of joint reinforcement or reinforced bond beams, effectively limits crack width in concrete masonry walls. As indicated previously, as the level of reinforcement increases and as the spacing of the reinforcement decreases, cracking becomes more uniformly distributed and crack width decreases. For this reason, a minimal amount of horizontal reinforcement is needed when utilizing the NCMA recommended maximum control joint spacings (refs. 3 & 4).
Walls in high seismic areas with a relatively large amount of horizontal reinforcement may not require control joints, as the reinforcement alone reduces the width of shrinkage cracks to a size that can be treated effectively with water repellent coatings. Experience has shown that this can be accomplished in walls with at least 0.2% of horizontal reinforcement (ref. 3). See Table 1 for the size and spacing of reinforcement to meet this criteria.
Table 1—Maximum Spacing of Horizontal Reinforcement to Meet the Criteria As > 0.002An
References
- Allowable Stress Design of Concrete Masonry Based on the 2012 IBC & 2011 MSJC, TEK 14-7C. National Concrete Masonry Association, 2013.
- Building Code Requirements for Masonry Structures, ACI 530-05/ASCE 6-05/TMS 402-05. Reported by the Masonry Standards Joint Committee, 2005.
- Control Joints for Concrete Masonry Walls – Alternative Engineered Method, TEK 10-3. National Concrete Masonry Association, 2003.
- Control Joints for Concrete Masonry Walls – Empirical Method, TEK 10-2C. National Concrete Masonry Association, 2010.
- Concrete Masonry Walls for Metal Buildings, TR-149. National Concrete Masonry Association, 1996.
- Crack Control for Concrete Brick and Other Concrete Masonry Veneers, TEK 10-4. National Concrete Masonry Association, 2001.
- Measuring Shrinkage of Concrete Block – A Comparison of Test Methods, E.L. Saxer and H.T. Toennies, Pages 988-1004, 1957.
- Standard Specification for Loadbearing Concrete Masonry Units, ASTM C90-03. ASTM International, 2003.
- Strength Design Provisions for Concrete Masonry, TEK 14-4B. National Concrete Masonry Association, 2008.
NCMA TEK 10-01A, Revised 2005.
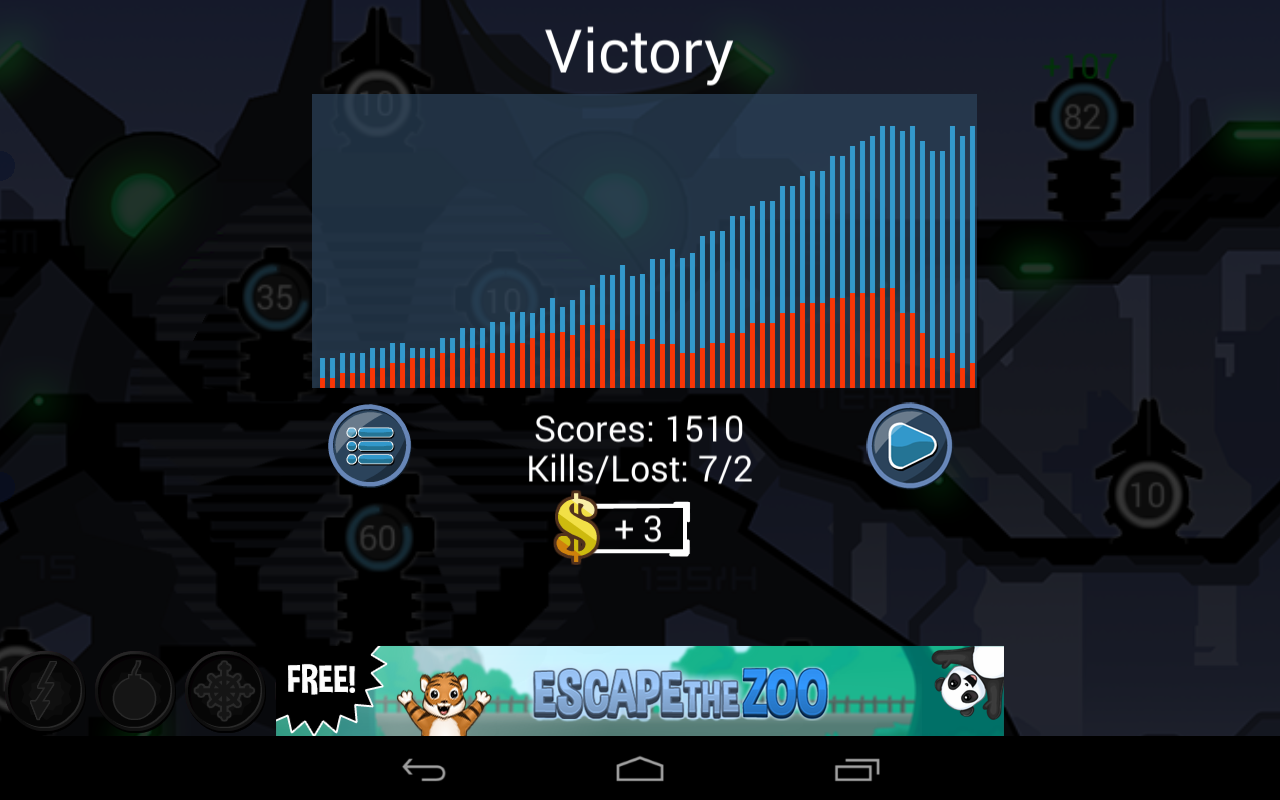
Although mortar, grout, and concrete masonry units are all concrete products, unit shrinkage has been shown to be the predominate indicator of the overall wall shrinkage principally due to the fact that it represents the largest portion of the wall. Therefore, the shrinkage properties of the unit alone are typically used to establish design criteria for crack control.
For an individual unit, the amount of drying shrinkage is influenced by the wetness of the unit at the time of placement as well as the characteristics and amount of cementitious materials, the type of aggregate, consolidation, and curing. Specifically, drying shrinkage is influenced in the following ways:
- walls constructed with 'wet' units will experience more drying shrinkage than drier units ;
- increases in cement content increase drying shrinkage;
- aggregates that are susceptible to volume change due to moisture content will result in increased shrinkage; and
- units that have undergone at least one drying cycle will not undergo as much shrinkage in subsequent drying cycles (ref. 7).
Typical drying shrinkage coefficients range from 0.0002 to 0.00045 in./in. (mm/mm) or 0.24 to 0.54 in. (6.1 to 13.7 mm) in 100 ft (30.48 m).
Temperature Changes
Concrete masonry movement has been shown to be linearly proportional to temperature change. The coefficient of thermal movement normally used in design is 0.0000045 in./in./°F (0.0000081 mm/mm/°C) (ref. 2). Actual values may range from 0.0000025 to 0.0000055 in./in./°F (0.0000045 to 0.0000099 mm/ mm/°C) depending mainly on the type of aggregate used in the unit. The actual change in temperature is, of course, determined by geographical location, wall exposure, and color.
Music - hearts of iron iv: sabaton soundtrack vol. 2 download for mac. As an example, a wall constructed during 70°F (21°C) weather and subjected to a minimum temperature of 0°F (-18°C) results in a shortening of about 0.38 in. (9.7 mm) in a 100 foot (30.48 m) long wall using the 0.0000045 in./in./°F (0.0000081 mm/mm/°C) coefficient.
Carbonation
Carbonation is an irreversible reaction between cementitious materials and carbon dioxide in the atmosphere that occurs slowly over a period of several years. Since there currently is no standard test method for carbonation shrinkage, it is suggested that a value of 0.00025 in./in. (mm/mm) be used. This results in a shortening of 0.3 in. (7.6 mm) in a 100 foot (30.48 m) long wall.
Restraint
As previously mentioned, the above phenomenon produce movement in the wall. When external restraint is provided that resists this movement, the result is tension within the wall and a corresponding potential for cracking. Typically, concrete masonry walls are restrained along the bottom of the wall (mainly by the foundation) with partial restraint along the top of the wall. The ends of the typical concrete masonry wall panel may be partially restrained by pilasters or wall intersections, but this partial restraint usually does not significantly alter the wall's cracking potential. Exceptions to the typical restraint condition include cantilevered walls which are restrained along their base, but free (unrestrained) at the top. It is conservative to base general crack control design criteria on a condition of restraint along the top and bottom of the wall.
Differential Movement
Various building materials may react differently to changes in temperature, moisture, or structural loading. Any time materials with different properties are combined in a wall system, a potential exists for cracking due to differential movement. With concrete masonry construction, two materials in particular should be considered: clay brick and structural steel.
Differential movement between clay brick and concrete masonry must be considered when the two are attached since concrete masonry has an overall tendency to shrink while clay brick masonry tends to expand. These differential movements may cause cracking, especially in composite construction and in walls that incorporate brick and block in the same wythe.
Composite walls are multi-wythe walls designed to act structurally, as a single unit in resisting applied loads. The wythes are typically bonded together using wall ties at prescribed intervals to assure adequate load transfer. When the composite wall includes a clay brick wythe bonded to a concrete masonry wythe, ladder-type joint reinforcement, or box ties are used to provide some degree of lateral movement between wythes. In addition, expansion joints are installed in the clay brick wythe to coincide with control joints in the concrete masonry wythe.
When clay brick is used as an accent band in a concrete masonry wall, or vice-versa, the differential movement of the two materials may result in cracking unless provisions are made to accommodate the movement. To reduce cracking, slip planes between the band and the surrounding wall, horizontal reinforcement or more frequent control joints or a combination thereof can be used to control cracking. See Crack Control for Concrete Brick and Other Concrete Masonry Veneers (ref. 6) for more information on these approaches.
Thermal movement differences also need to be taken into consideration when using masonry in conjunction with structural steel. In addition to differences in thermal coefficients, steel shapes typically have a much higher surface area to volume ratio and tend to react to changes in temperature more quickly. This is normally accommodated with slotted and flexible connections. Concrete Masonry Walls for Metal Buildings (ref. 5) provides more detailed information on this subject.
Excessive Deflection
As walls and beams deflect under structural loads, cracking may occur. Additionally, deflection of supporting members can induce cracks in masonry elements. To reduce the potential for cracking, the following alternatives are available:
- adding reinforcing steel into the masonry to cross the expected cracks and to limit the width of the cracks,
- limiting the deflection of members providing vertical support of unreinforced masonry to acceptable levels (less than or equal to l/600 nor more than 0.3 in. (7.6 mm) due to dead load and live load when supporting unreinforced masonry) (ref. 2), and;
- utilizing movement joints to effectively panelize the masonry so that it can articulate with the deflected shape of the supporting member.
Structural Overload
All wall systems are subject to potential cracking from externally applied design loads due to wind, soil pressure or seismic forces. Cracking due to these sources is controlled by applying appropriate structural design criteria such as allowable stress design or strength design. These criteria are discussed in detail in Allowable Stress Design of Concrete Masonry and Strength Design Provisions for Concrete Masonry (refs. 1 and 9).
Settlement
Differential settlement occurs when portions of the supporting foundation subside due to weak or improperly compacted foundation soils. Foundation settlement typically causes a stair-step crack along the mortar joints in the settled area as shown in Figure 1. Preventing settlement cracking depends on a realistic evaluation of soil bearing capacity, and on proper footing design and construction.
Dragon age origins korcari wilds map. Footings should be placed on undisturbed native soil, unless this soil is unsuitable, weak, or soft. Unsuitable soil should be removed and replaced with compacted soil, gravel, or concrete. Similarly, tree roots, construction debris, and ice should be removed prior to placing footings. Adding reinforcement in foundations can also lessen the effects of differential settlement.
CRACK CONTROL STRATEGIES
In addition to the proper design strategies discussed above for structural capacity and differential movement, the following recommendations can be applied to limit cracking in concrete masonry walls.
Material Properties
Traditionally, crack control in concrete masonry has relied on specifying concrete masonry units with a low moisture content, using horizontal reinforcement, and using control joints to accommodate movement. Prior to the 2000 edition of ASTM C90 (ref. 8), low moisture content was specified by requiring a Type I moisture controlled unit. The intent was to provide designers an assurance of units with lower moisture content to minimize potential shrinkage cracking. However, there are several limitations to relying on moisture content alone since there are other factors that influence shrinkage which are not accounted for by specifying a Type I unit. Additionally, Type I units were not always inventoried by concrete masonry manufacturers. Most importantly, Type I units needed to be kept protected until placed in the wall, which was proven to be difficult on some projects. Because of the above problems associated with the Type I specification, ASTM removed the designations of Type I, Moisture-Controlled Units and Type II, Nonmoisture Controlled Units from the standard.
Due to removal of the unit type designations from ASTM C90, two methods of determining control joint spacings have been devised irrespective of unit type: 1). Empirical crack control criteria which is based on successful, historical performance over many years in various geographic conditions and 2). Engineered crack control criteria based on a Crack Control Coefficient (CCC) that includes the combined effects of movement due to drying shrinkage, carbonation shrinkage, and contraction due to temperature change. The first is presented in NCMA TEK 10-2C, Control Joints for Concrete Masonry Walls – Empirical Method (ref. 4) and the second in TEK 10-3 Control Joints for Concrete Masonry Walls – Alternative Engineered Method (ref. 3). The empirical method is the most commonly used method and is applicable to most conventional building types. The engineered method is generally used only when unusual conditions are encountered such as dark colored units in climates with large temperature swings.
Control Joints
Control joints are essentially vertical separations built into the wall to reduce restraint and permit longitudinal movement. Because shrinkage cracks in concrete masonry are an aesthetic rather than a structural concern, control joints are typically only required in walls where shrinkage cracking may detract from the appearance or where water penetration may occur. TEK 10-2C (ref. 4) provides much more detailed information on control joint details, types and locations.
Reinforcement to Limit Crack Width
In addition to external restraint, reinforcement causes some internal restraint within the wall. Reinforcement responds to temperature changes with corresponding changes in length; however, reinforcement does not undergo volumetric changes due to moisture changes or carbonation. Consequently, as the wall shrinks, the reinforcement undergoes elastic shortening (strain) which results in compressive stress in the steel. Correspondingly, the surrounding masonry offsets this compression by tension. At the point when the masonry cracks and tries to open, the stress in the reinforcement turns to tension and acts to limit the width of the crack by holding it closed.
The net effect is that reinforcement controls crack width by causing a greater number (frequency) of cracks to occur. As the horizontal reinforcement ratio (cross-sectional area of horizontal steel vs. vertical cross-sectional area of masonry) increases, crack width decreases. Smaller sized reinforcement at closer spacings is more effective than larger reinforcement at wider spacings, although horizontal reinforcement at spacings up to 144 in. (3658 mm) is considered effective in controlling crack widths in some areas.
Studies have shown that reinforcement, either in the form of joint reinforcement or reinforced bond beams, effectively limits crack width in concrete masonry walls. As indicated previously, as the level of reinforcement increases and as the spacing of the reinforcement decreases, cracking becomes more uniformly distributed and crack width decreases. For this reason, a minimal amount of horizontal reinforcement is needed when utilizing the NCMA recommended maximum control joint spacings (refs. 3 & 4).
Walls in high seismic areas with a relatively large amount of horizontal reinforcement may not require control joints, as the reinforcement alone reduces the width of shrinkage cracks to a size that can be treated effectively with water repellent coatings. Experience has shown that this can be accomplished in walls with at least 0.2% of horizontal reinforcement (ref. 3). See Table 1 for the size and spacing of reinforcement to meet this criteria.
Table 1—Maximum Spacing of Horizontal Reinforcement to Meet the Criteria As > 0.002An
References
- Allowable Stress Design of Concrete Masonry Based on the 2012 IBC & 2011 MSJC, TEK 14-7C. National Concrete Masonry Association, 2013.
- Building Code Requirements for Masonry Structures, ACI 530-05/ASCE 6-05/TMS 402-05. Reported by the Masonry Standards Joint Committee, 2005.
- Control Joints for Concrete Masonry Walls – Alternative Engineered Method, TEK 10-3. National Concrete Masonry Association, 2003.
- Control Joints for Concrete Masonry Walls – Empirical Method, TEK 10-2C. National Concrete Masonry Association, 2010.
- Concrete Masonry Walls for Metal Buildings, TR-149. National Concrete Masonry Association, 1996.
- Crack Control for Concrete Brick and Other Concrete Masonry Veneers, TEK 10-4. National Concrete Masonry Association, 2001.
- Measuring Shrinkage of Concrete Block – A Comparison of Test Methods, E.L. Saxer and H.T. Toennies, Pages 988-1004, 1957.
- Standard Specification for Loadbearing Concrete Masonry Units, ASTM C90-03. ASTM International, 2003.
- Strength Design Provisions for Concrete Masonry, TEK 14-4B. National Concrete Masonry Association, 2008.
NCMA TEK 10-01A, Revised 2005.
NCMA and the companies disseminating this technical information disclaim any and all responsibility and liability for the accuracy and the application of the information contained in this publication.
E. Dillon & Company
- Swords Creek
- 800-234-8970
If you have questions about specific products or services we provide, please don't hesitate to contact us.
INTRODUCTION
Cracks in buildings and building materials normally result from restrained movement. This movement may originate within the material, as with volume changes due to moisture loss or acquisition, temperature expansion or contraction, or may result from movements of adjacent or supporting materials, such as deflection of beams or slabs. In many cases, movement is inevitable and must be accommodated or controlled.
Designing for effective crack control requires an understanding of the sources of stress which may cause cracking. It would be a simple matter to prevent cracking if there were only one variable. However, prevention is made more difficult by the fact that cracking often results from a combination of sources.
CAUSES OF CRACKING
There are a variety of potential causes of cracking. Understanding the cause of potential cracking allows the designer to incorporate appropriate design procedures to control it. The most common causes of cracking in concrete masonry are shown in Figure 1 and are discussed below.
Figure 1 – Proper Design Can Avert Cracking of These Types
Shrinkage/Restraint
Cracking resulting from shrinkage can occur in concrete masonry walls because of drying shrinkage, temperature fluctuations, and carbonation. These cracks occur when masonry panels are restrained from moving.
Drying Shrinkage
Concrete products are composed of a matrix of aggregate particles coated by cement which bonds them together. Once the concrete sets, this cementitious-coated aggregate matrix expands with increasing moisture content and contracts (shrinks) with decreasing moisture content. Drying shrinkage is therefore a function of change in moisture content.
Although mortar, grout, and concrete masonry units are all concrete products, unit shrinkage has been shown to be the predominate indicator of the overall wall shrinkage principally due to the fact that it represents the largest portion of the wall. Therefore, the shrinkage properties of the unit alone are typically used to establish design criteria for crack control.
For an individual unit, the amount of drying shrinkage is influenced by the wetness of the unit at the time of placement as well as the characteristics and amount of cementitious materials, the type of aggregate, consolidation, and curing. Specifically, drying shrinkage is influenced in the following ways:
- walls constructed with 'wet' units will experience more drying shrinkage than drier units ;
- increases in cement content increase drying shrinkage;
- aggregates that are susceptible to volume change due to moisture content will result in increased shrinkage; and
- units that have undergone at least one drying cycle will not undergo as much shrinkage in subsequent drying cycles (ref. 7).
Typical drying shrinkage coefficients range from 0.0002 to 0.00045 in./in. (mm/mm) or 0.24 to 0.54 in. (6.1 to 13.7 mm) in 100 ft (30.48 m).
Temperature Changes
Concrete masonry movement has been shown to be linearly proportional to temperature change. The coefficient of thermal movement normally used in design is 0.0000045 in./in./°F (0.0000081 mm/mm/°C) (ref. 2). Actual values may range from 0.0000025 to 0.0000055 in./in./°F (0.0000045 to 0.0000099 mm/ mm/°C) depending mainly on the type of aggregate used in the unit. The actual change in temperature is, of course, determined by geographical location, wall exposure, and color.
As an example, a wall constructed during 70°F (21°C) weather and subjected to a minimum temperature of 0°F (-18°C) results in a shortening of about 0.38 in. (9.7 mm) in a 100 foot (30.48 m) long wall using the 0.0000045 in./in./°F (0.0000081 mm/mm/°C) coefficient.
Carbonation
Carbonation is an irreversible reaction between cementitious materials and carbon dioxide in the atmosphere that occurs slowly over a period of several years. Since there currently is no standard test method for carbonation shrinkage, it is suggested that a value of 0.00025 in./in. (mm/mm) be used. This results in a shortening of 0.3 in. (7.6 mm) in a 100 foot (30.48 m) long wall.
Restraint
As previously mentioned, the above phenomenon produce movement in the wall. When external restraint is provided that resists this movement, the result is tension within the wall and a corresponding potential for cracking. Typically, concrete masonry walls are restrained along the bottom of the wall (mainly by the foundation) with partial restraint along the top of the wall. The ends of the typical concrete masonry wall panel may be partially restrained by pilasters or wall intersections, but this partial restraint usually does not significantly alter the wall's cracking potential. Exceptions to the typical restraint condition include cantilevered walls which are restrained along their base, but free (unrestrained) at the top. It is conservative to base general crack control design criteria on a condition of restraint along the top and bottom of the wall.
Differential Movement
Various building materials may react differently to changes in temperature, moisture, or structural loading. Any time materials with different properties are combined in a wall system, a potential exists for cracking due to differential movement. With concrete masonry construction, two materials in particular should be considered: clay brick and structural steel.
Differential movement between clay brick and concrete masonry must be considered when the two are attached since concrete masonry has an overall tendency to shrink while clay brick masonry tends to expand. These differential movements may cause cracking, especially in composite construction and in walls that incorporate brick and block in the same wythe.
Composite walls are multi-wythe walls designed to act structurally, as a single unit in resisting applied loads. The wythes are typically bonded together using wall ties at prescribed intervals to assure adequate load transfer. When the composite wall includes a clay brick wythe bonded to a concrete masonry wythe, ladder-type joint reinforcement, or box ties are used to provide some degree of lateral movement between wythes. In addition, expansion joints are installed in the clay brick wythe to coincide with control joints in the concrete masonry wythe.
When clay brick is used as an accent band in a concrete masonry wall, or vice-versa, the differential movement of the two materials may result in cracking unless provisions are made to accommodate the movement. To reduce cracking, slip planes between the band and the surrounding wall, horizontal reinforcement or more frequent control joints or a combination thereof can be used to control cracking. See Crack Control for Concrete Brick and Other Concrete Masonry Veneers (ref. 6) for more information on these approaches.
Thermal movement differences also need to be taken into consideration when using masonry in conjunction with structural steel. In addition to differences in thermal coefficients, steel shapes typically have a much higher surface area to volume ratio and tend to react to changes in temperature more quickly. This is normally accommodated with slotted and flexible connections. Concrete Masonry Walls for Metal Buildings (ref. 5) provides more detailed information on this subject.
Excessive Deflection
As walls and beams deflect under structural loads, cracking may occur. Additionally, deflection of supporting members can induce cracks in masonry elements. To reduce the potential for cracking, the following alternatives are available:
- adding reinforcing steel into the masonry to cross the expected cracks and to limit the width of the cracks,
- limiting the deflection of members providing vertical support of unreinforced masonry to acceptable levels (less than or equal to l/600 nor more than 0.3 in. (7.6 mm) due to dead load and live load when supporting unreinforced masonry) (ref. 2), and;
- utilizing movement joints to effectively panelize the masonry so that it can articulate with the deflected shape of the supporting member.
Structural Overload
All wall systems are subject to potential cracking from externally applied design loads due to wind, soil pressure or seismic forces. Cracking due to these sources is controlled by applying appropriate structural design criteria such as allowable stress design or strength design. These criteria are discussed in detail in Allowable Stress Design of Concrete Masonry and Strength Design Provisions for Concrete Masonry (refs. 1 and 9).
Settlement
Differential settlement occurs when portions of the supporting foundation subside due to weak or improperly compacted foundation soils. Foundation settlement typically causes a stair-step crack along the mortar joints in the settled area as shown in Figure 1. Preventing settlement cracking depends on a realistic evaluation of soil bearing capacity, and on proper footing design and construction.
Footings should be placed on undisturbed native soil, unless this soil is unsuitable, weak, or soft. Unsuitable soil should be removed and replaced with compacted soil, gravel, or concrete. Similarly, tree roots, construction debris, and ice should be removed prior to placing footings. Adding reinforcement in foundations can also lessen the effects of differential settlement.
CRACK CONTROL STRATEGIES
In addition to the proper design strategies discussed above for structural capacity and differential movement, the following recommendations can be applied to limit cracking in concrete masonry walls.
Material Properties
Traditionally, crack control in concrete masonry has relied on specifying concrete masonry units with a low moisture content, using horizontal reinforcement, and using control joints to accommodate movement. Prior to the 2000 edition of ASTM C90 (ref. 8), low moisture content was specified by requiring a Type I moisture controlled unit. The intent was to provide designers an assurance of units with lower moisture content to minimize potential shrinkage cracking. However, there are several limitations to relying on moisture content alone since there are other factors that influence shrinkage which are not accounted for by specifying a Type I unit. Additionally, Type I units were not always inventoried by concrete masonry manufacturers. Most importantly, Type I units needed to be kept protected until placed in the wall, which was proven to be difficult on some projects. Because of the above problems associated with the Type I specification, ASTM removed the designations of Type I, Moisture-Controlled Units and Type II, Nonmoisture Controlled Units from the standard.
Control Craft 3
Due to removal of the unit type designations from ASTM C90, two methods of determining control joint spacings have been devised irrespective of unit type: 1). Empirical crack control criteria which is based on successful, historical performance over many years in various geographic conditions and 2). Engineered crack control criteria based on a Crack Control Coefficient (CCC) that includes the combined effects of movement due to drying shrinkage, carbonation shrinkage, and contraction due to temperature change. The first is presented in NCMA TEK 10-2C, Control Joints for Concrete Masonry Walls – Empirical Method (ref. 4) and the second in TEK 10-3 Control Joints for Concrete Masonry Walls – Alternative Engineered Method (ref. 3). The empirical method is the most commonly used method and is applicable to most conventional building types. The engineered method is generally used only when unusual conditions are encountered such as dark colored units in climates with large temperature swings.
Control Joints
Control joints are essentially vertical separations built into the wall to reduce restraint and permit longitudinal movement. Because shrinkage cracks in concrete masonry are an aesthetic rather than a structural concern, control joints are typically only required in walls where shrinkage cracking may detract from the appearance or where water penetration may occur. TEK 10-2C (ref. 4) provides much more detailed information on control joint details, types and locations.
Control Craft 3 Hacked
Reinforcement to Limit Crack Width
In addition to external restraint, reinforcement causes some internal restraint within the wall. Reinforcement responds to temperature changes with corresponding changes in length; however, reinforcement does not undergo volumetric changes due to moisture changes or carbonation. Consequently, as the wall shrinks, the reinforcement undergoes elastic shortening (strain) which results in compressive stress in the steel. Correspondingly, the surrounding masonry offsets this compression by tension. At the point when the masonry cracks and tries to open, the stress in the reinforcement turns to tension and acts to limit the width of the crack by holding it closed.
The net effect is that reinforcement controls crack width by causing a greater number (frequency) of cracks to occur. As the horizontal reinforcement ratio (cross-sectional area of horizontal steel vs. vertical cross-sectional area of masonry) increases, crack width decreases. Smaller sized reinforcement at closer spacings is more effective than larger reinforcement at wider spacings, although horizontal reinforcement at spacings up to 144 in. (3658 mm) is considered effective in controlling crack widths in some areas.
Studies have shown that reinforcement, either in the form of joint reinforcement or reinforced bond beams, effectively limits crack width in concrete masonry walls. As indicated previously, as the level of reinforcement increases and as the spacing of the reinforcement decreases, cracking becomes more uniformly distributed and crack width decreases. For this reason, a minimal amount of horizontal reinforcement is needed when utilizing the NCMA recommended maximum control joint spacings (refs. 3 & 4).
Walls in high seismic areas with a relatively large amount of horizontal reinforcement may not require control joints, as the reinforcement alone reduces the width of shrinkage cracks to a size that can be treated effectively with water repellent coatings. Experience has shown that this can be accomplished in walls with at least 0.2% of horizontal reinforcement (ref. 3). See Table 1 for the size and spacing of reinforcement to meet this criteria.
Table 1—Maximum Spacing of Horizontal Reinforcement to Meet the Criteria As > 0.002An
References
- Allowable Stress Design of Concrete Masonry Based on the 2012 IBC & 2011 MSJC, TEK 14-7C. National Concrete Masonry Association, 2013.
- Building Code Requirements for Masonry Structures, ACI 530-05/ASCE 6-05/TMS 402-05. Reported by the Masonry Standards Joint Committee, 2005.
- Control Joints for Concrete Masonry Walls – Alternative Engineered Method, TEK 10-3. National Concrete Masonry Association, 2003.
- Control Joints for Concrete Masonry Walls – Empirical Method, TEK 10-2C. National Concrete Masonry Association, 2010.
- Concrete Masonry Walls for Metal Buildings, TR-149. National Concrete Masonry Association, 1996.
- Crack Control for Concrete Brick and Other Concrete Masonry Veneers, TEK 10-4. National Concrete Masonry Association, 2001.
- Measuring Shrinkage of Concrete Block – A Comparison of Test Methods, E.L. Saxer and H.T. Toennies, Pages 988-1004, 1957.
- Standard Specification for Loadbearing Concrete Masonry Units, ASTM C90-03. ASTM International, 2003.
- Strength Design Provisions for Concrete Masonry, TEK 14-4B. National Concrete Masonry Association, 2008.
NCMA TEK 10-01A, Revised 2005.
NCMA and the companies disseminating this technical information disclaim any and all responsibility and liability for the accuracy and the application of the information contained in this publication.